A Customized 3D-Printed Bolus for High-Risk Breast Cancer with Skin Infiltration: A Pilot Study
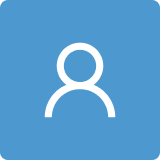
Round 1
Reviewer 1 Report
Comments and Suggestions for AuthorsThe paper investigates the development and application of 3D-printed bolus materials in radiotherapy for high-risk breast cancer. It emphasizes the customization potential of these boluses, which can closely conform to a patient's unique anatomy, potentially leading to more accurate and effective radiation dose delivery. I am looking forward for the better version of your manuscript.
Comments for author File: Comments.pdf
Author Response
Comments 1. Dosimetric results: in this part, dosimetric comparison between the standard bolus and 3D-printed bolus was important to check the skin irradiation dosage and distribution. But I did not see the results.
Response 1. In our study we didn’t compare standard and 3D-printed bolus dosimetrically, because it is only a pilot study to assess that the use of a 3D printed bolus in clinical practice is adequate to guarantee enough surface dose, without significant toxicity for the patient. We focused on detailing the procedure to print a 3D bolus for clinical routine; regarding the results we highlighted clinical outcomes rather than dosimetric results to better meet the journal target. A detailed dosimetric comparison could be very interesting in further studies with larger number of patients, with sufficient statistical confidence. However, as reported in the discussion section, in literature there are already many dosimetric studies comparing standard and customized boluses.
Comments 2. Sample Size: The study might have a limited sample size or lack long-term follow-up survival data, which can restrict the generalizability of the findings.
Response 2. The study is only a pilot study with a preliminary observational purpose, however it could represent the basis of a future study with a larger number of patients. The limited sample size is mainly due to the difficulty to recruit a large number of high-risk breast cancer patients with skin infiltration. Moreover, the editor suggested us to classify the paper as Case Report.
Comments 3. Technology Accessibility: There may be limitations in the accessibility of 3D printing technology across different healthcare settings, which could hinder widespread adoption. Also, the biological safety and biocompatibility of the 3D-printed materials should be discussed.
Response 3. To introduce 3D printing technology into a radiotherapy unit it is only necessary to provide a professional 3D printer and printing materials (PLA filaments), both readily available on market at relatively low costs (see point n. 4). We added the sentence at line 116: “PLA is one of the most widely used polymers in clinical applications today, due to its favourable biocompatibility and to its innocuous degradation products that can safely coexist with biological systems [9]. Moreover, PLA is a readily available material at relatively low cost with respect to standard gel bolus”.
[9] da Silva, D.; Kaduri, M.; Poley, M.; Adir, O.; Krinsky, N.; Shainsky-Roitman, J.; Schroeder, A. Biocompatibility, biodegradation and excretion of polylactic acid (PLA) in medical implants and theranostic systems. Chem Eng J. 2018 May 15;340:9-14. doi: 10.1016/j.cej.2018.01.010.
Comments 4. Cost Analysis: The paper may not thoroughly address the cost-effectiveness of using 3D-printed boluses compared to conventional methods. I would suggest the authors to Include a Comprehensive Cost-Benefit Analysis, to determine the overall cost-effectiveness, potentially integrating this analysis into the broader healthcare system's financial considerations.
Response 4. We added the sentence at line 300: “Regarding cost analysis, the investment of a professional device could be widely amortized over the years, considering that the cost of a PLA bolus filled with ultrasound gel is around 6-10$”.
Comments 5. Clinical Workflow Integration: The paper might not fully explore the integration of 3D printing into existing clinical workflows, including the time and training required for clinical doctors and medical physicist.
Response 5. We modified the sentences from line 285 as follows: “This provides significant advantages for the patient. However, the whole printing process can be time-consuming and cumbersome to manage for the operator. This represents one of the main limitations for the integration of 3D printing in a clinical workflow. In fact, the procedure to create a single 3D printed bolus is particularly detailed and should be clearly drafted to train technical and medical physics staff. Moreover, the printing process could last up to 10-12 h depending on printer model and bolus size. In addition, technical issues could occur aborting the whole printing process, thus the device should be printed in advance with a sufficient margin of time from the treatment start”.
Reviewer 2 Report
Comments and Suggestions for AuthorsThis paper describes work to develop a method for 3D printing personalized bolus for breast cancer patients and evaluating clinical outcome in seven patients. In general the paper is well written. Many other institutions have used customized bolus 3D printed in-house or with a third party vendor. The novelty of this technique is to fill a hollow bolus shell with ultrasound gel material. Seven patients is not a large population to evaluate whether this bolus technique is a vast improvement over other 3D printed bolus with varying materials, Superflab, brass mesh bolus, or other methods especially since one of the patients died and another was lost to followup leaving only five test subjects. It would have been nice if the authors had completed a retrospective study of like patients using non-customized bolus as a control comparison for post-treatment symptoms such as pain, erythema, hyperpigmentation, and telangiectasia. This would help determine if side effects would be worse due to the higher uniform skin dose.
Specific comments:
Line 104: It is unclear how the authors determined where the MOSFETs would be placed on the phantom and on the patients.
Paragraph, starting on line 117: It is unclear how the bolus was filled with the gel material, then sealed to prevent leaking over the course of treatment.
Line 133: I am not sure what is meant by “half using medial and half using external entries.” Please clarify what is meant here? I am guessing that a half beam block was used, not sure.
Section 2.6 and 2.7: Were quality assurance CBCTs taken to verify that there were no air gaps between phantom/patient skin surface and bolus?
Section 3.1: The material section describe two MOSFET dosimeters (standard and micro), but it is unclear if the authors ended up using both or one specific model for the clinical measurements. Were there differences between the standard and micro dosimeters? If so, what were the differences?
Section 3.2: For patients with increased erythema, were these the patients that had the worse symptoms at long-term follow up? Was the observed acute erythema and pain uniform over the entire breast or only in specific smaller regions?
Author Response
Comments 1. Line 104: It is unclear how the authors determined where the MOSFETs would be placed on the phantom and on the patients.
Response 1. We modified the sentences at lines 104-110 as follows: “Five small spherical ROIs were freehand contoured on patients CTs at skin-bolus interface in the positions where the dose was planned to be evaluated. The five ROIs were subtracted from the bolus ROI generating five holes at its inner surface, serving as suitable housing for MOSFET dosimeters positioning (Figure 2). Thus, for the following dosimetric evaluation the position of the MOSFETs at bolus inner surface corresponded to the ROIs contoured on the TPS. Figure 1 shows the five chosen positions: A cranial, B central, C caudal, D medial, and E external”.
Comments 2. Paragraph, starting on line 117: It is unclear how the bolus was filled with the gel material, then sealed to prevent leaking over the course of treatment.
Response 2. We added the sentence at line 127: “Bolus walls were drilled with small holes and filled with gel using a syringe, then sealed with glue to prevent material leakage over the course of treatment”.
Comments 3. Line 133: I am not sure what is meant by “half using medial and half using external entries.” Please clarify what is meant here? I am guessing that a half beam block was used, not sure.
Response 3. We didn’t mean that a half beam block was used, we just wanted to say that we used, for example, two medial and two external beams in a total of four beams. We simplified the sentence as follows: “The treatment technique employed for both the phantom and patients involved four to six intensity modulated (IMRT) tangential beams, with medial or external entries, in sliding window delivery mode”.
Comments 4. Section 2.6 and 2.7: Were quality assurance CBCTs taken to verify that there were no air gaps between phantom/patient skin surface and bolus?
Response 4. We already specified that at line 153: “A cone beam CT (CBCT) was acquired before each treatment delivery, to verify the correct patient positioning and bolus fitting”, at line 181: “On the basis of daily CBCT acquisition, we observed that the positioning of the 3D bolus is reproducible and its shape better conforms to the skin, visibly reducing air gaps”, and in Figure 5 caption: “CT scan of a patient with prosthesis and standard gel bolus (a) and CBCT acquired before radiation delivery in the same patient (b). 3D bolus fits the skin without relevant air gaps”.
Comments 5. Section 3.1: The material section describe two MOSFET dosimeters (standard and micro), but it is unclear if the authors ended up using both or one specific model for the clinical measurements. Were there differences between the standard and micro dosimeters? If so, what were the differences?
Response 5. We modified the sentence at line 162 as follows: “Specifically, a set of dosimeters composed of three standard model (TN-502RD-H) and two microMOSFET model (TN-502RDM-H) was used”. The difference between the two models consists only on the different size of the dosimeter, as specified at line 170.
Comments 6. Section 3.2: For patients with increased erythema, were these the patients that had the worse symptoms at long-term follow up? Was the observed acute erythema and pain uniform over the entire breast or only in specific smaller regions?
Response 6. We modified the sentence at line 229 as follows: “Patients who developed erythema G2 experienced worse late toxicities. After one year, G2 local pain persisted in the patient with prosthesis uniformly on the entire irradiated chest area. One patient had G1 telangiectasia more evident at the level of the upper quadrants of the chest, one patient showed G1 patchy hyperpigmentation and 2 patients had no relevant toxicity”.
Round 2
Reviewer 1 Report
Comments and Suggestions for AuthorsThank you very much for your efforts on your work.