Antioxidative, Antiproliferative and Antimicrobial Activities of Phenolic Compounds from Three Myrcia Species
Abstract
: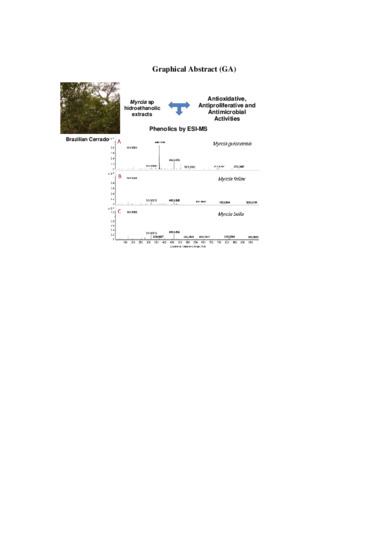
Graphical Abstract
1. Introduction
2. Results and Discussion
3. Experimental Section
3.1. Drugs and Chemicals
3.2. Collection and Preparation of Plant Material
3.3. Polyphenol and Flavonoid Contents
3.4. ESI(−)-Q-TOF-MS Analysis
3.5. Determination of DPPH Radical Scavenging
3.6. In Vitro Antiproliferative Activity Assay
3.7. Minimum Inhibitory Concentration
3.8. Statistical Analysis
Author Contributions
Acknowledgments
Conflicts of Interest
References
- Costa, M.F.; Jesus, T.I.B.; Lopes, R.P.; Angolini, C.F.; Montagnolli, A.; Gomes, L.P.; Pereira, G.S.; Ruiz, A.L.; Carvalho, J.E.; Eberlin, M.N.; et al. Eugenia aurata and Eugenia punicifolia HBK Inhibit Inflammatory Response by Reducing Neutrophil Adhesion, Degranulation and NET Release. BMC Complement. Altern. Med. 2016, 16, 1–10. [Google Scholar] [CrossRef] [PubMed]
- Cascaes, M.M.; Guilhon, G.M.S.P.; Andrade, E.H.A.; Zoghbi, M.G.B.; Santos, L.S. Constituents and Pharmacological Activities of Myrcia (Myrtaceae): A Review of an Aromatic and Medicinal Group of Plants. Int. J. Mol. Sci. 2015, 16, 23881–23904. [Google Scholar] [CrossRef] [PubMed]
- Rice-Evans, C.A.; Miller, N.J.; Paganga, G. Structure-Antioxidant Activity Relationships of Flavonoids and Phenolic Acids. Free Radic. Biol. Med. 1996, 20, 933–956. [Google Scholar] [CrossRef]
- Landete, J.M. Ellagitannins, Ellagic Acid and Their Derived Metabolites: A Review about Source, Metabolism, Functions and Health. Food Res. Int. 2011, 44, 1150–1160. [Google Scholar] [CrossRef]
- Moresco, H.H.; Pereira, M.; Bretanha, L.C.; Micke, G.A.; Pizzolatti, M.G.; Brighentea, I.M.C. Myricitrin as the Main Constituent of Two Species of Myrcia. J. Appl. Pharm. Sci. 2014, 4, 1–7. [Google Scholar]
- Da Silva Sá, F.A.; de Paula, J.A.M.; Dos Santos, P.A.; de Almeida Ribeiro Oliveira, L.; de Almeida Ribeiro Oliveira, G.; Lião, L.M.; de Paula, J.R.; do Rosário Rodrigues Silva, M. Phytochemical Analysis and Antimicrobial Activity of Myrcia tomentosa (Aubl.) DC. Leaves. Molecules 2017, 22. [Google Scholar] [CrossRef] [PubMed]
- Nuengchamnong, N.; Ingkaninan, K. On-Line Characterization of Phenolic Antioxidants in Fruit Wines from Family Myrtaceae by Liquid Chromatography Combined with Electrospray Ionization Tandem Mass Spectrometry and Radical Scavenging Detection. LWT—Food Sci. Technol. 2009, 42, 297–302. [Google Scholar] [CrossRef]
- Carvajal, P.C.; Coppo, E.; Di Lorenzo, A.; Gozzini, D.; Bracco, F.; Zanoni, G.; Nabavi, S.M.; Marchese, A.; Arciola, C.R.; Daglia, M. Chemical characterization and in vitro Antibacterial Activity of Myrcianthes hallii (O. Berg) McVaugh (Myrtaceae), a Traditional Plant Growing in Ecuador”. Materials 2016, 9, 454–468. [Google Scholar] [CrossRef] [PubMed]
- Hossain, M.B.; Rai, D.K.B.; Martin-Diana, N.P.; Barry-Ryan, A.B.C. Characterization of Phenolic Composition in Lamiaceae Spices by LC-ESI-MS/MS. J. Agric. Food Chem. 2010, 58, 10576–10581. [Google Scholar] [CrossRef] [PubMed]
- Oliveira, A.P.; Pereira, J.A.; Valentão, P.B.; Valentão, P.; Seabra, R.M.; Silva, B.M. Organic Acids Composition of Cydonia oblonga Miller Leaf. Food Chem. 2008, 111, 393–399. [Google Scholar] [CrossRef] [PubMed]
- Da Costa, M.F.; Galaverna, R.S.; Pudenzi, M.A.; Ruiz, A.L.T.G.; De Carvalho, J.E.; Eberlin, M.N.; Dos Santos, C. Profiles of Phenolic Compounds by FT-ICR MS and Antioxidative and Antiproliferative Activities of: Stryphnodendron obovatum Benth Leaf Extracts”. Anal. Methods 2016, 8, 6056–6063. [Google Scholar] [CrossRef]
- Lee, J.H.; Johnson, J.V.; Talcott, S.T. Identification of Ellagic Acid Conjugates and Other Polyphenolics in Muscadine Grapes by HPLC-ESI-MS. J. Agric. Food Chem. 2005, 53, 6003–6010. [Google Scholar] [CrossRef] [PubMed]
- Romani, A.; Campo, M.; Pinelli, P. HPLC/DAD/ESI-MS Analyses and Anti-Radical Activity of Hydrolyzable Tannins from Different Vegetal Species”. Food Chem. 2012, 130, 214–221. [Google Scholar] [CrossRef]
- Soong, Y.Y.; Barlow, P.J. Isolation and Structure Elucidation of Phenolic Compounds from Longan (Dimocarpus longan Lour.) Seed by High-Performance Liquid Chromatography-Electrospray Ionization Mass Spectrometry. J. Chromatogr. A 2005, 1085, 270–277. [Google Scholar] [CrossRef] [PubMed]
- Sandhu, A.K.; Gu, L. Antioxidant Capacity, Phenolic Content, and Profiling of Phenolic Compounds in the Seeds, Skin, and Pulp of Vitis rotundifolia (Muscadine Grapes) as Determined by HPLC-DAD-ESI-MSn”. J. Agric. Food Chem. 2010, 58, 4681–4692. [Google Scholar] [CrossRef] [PubMed]
- Dutra, R.P.; Abreu, B.V.; Cunha, M.S.; Batista, M.C.; Torres, L.M.; Nascimento, F.R.; Ribeiro, M.N.; Guerra, R.N. Phenolic Acids, Hydrolyzable Tannins, and Antioxidant Activity of Geopropolis from the Stingless Bee Melipona fasciculata Smith. J. Agric. Food Chem. 2014, 62, 2549–2557. [Google Scholar] [CrossRef] [PubMed]
- Wu, S.; Dastmalchi, K.; Long, C.; Kennelly, E.J. Metabolite Profiling of Jaboticaba (Myrciaria cauliflora) and Other Dark-Colored Fruit Juices. J. Agric. Food Chem. 2012, 60, 7513–7525. [Google Scholar] [CrossRef] [PubMed]
- Regueiro, J.; Sanchez-Gonzalez, C.; Vallverde-Queralt, A.; Simal-Gandara, J.; Lamuela-Raventós, R.M.; Izquierdo-Pulido, M. Comprehensive Identification of Walnut Polyphenols by Liquid Chromatography Coupled to Linear Ion Trap-Orbitrap Mass Spectrometry. Food Chem. 2014, 152, 340–348. [Google Scholar] [CrossRef] [PubMed]
- Fabre, N.; Rustan, I.; De Hoffmann, E.; Quetin-Leclercq, J. Determination of Flavone, Flavonol, and Flavanone Aglycones by Negative Ion Liquid Chromatography Electrospray Ion Trap Mass Spectrometry”. J. Am. Soc. Mass Spectrom. 2001, 12, 707–715. [Google Scholar] [CrossRef]
- Ma, Y.L.; Li, Q.M.; Van Den Heuvel, H.; Claeys, M. Characterization of Flavone and Flavonol Aglycones by Collision-Induced Dissociation Tandem Mass Spectrometry. Rapid Commun. Mass Spectrom. 1997, 11, 1357–1364. [Google Scholar] [CrossRef]
- Ablajan, K.; Zeper, A.; Xiao-Ya, S.; Jiu-Ming, H.; Rui-Ping, Z.; Jian-Gong, S. Structural Characterization of Flavonol 3,7-Di-O-Glycosides and Determination of the Glycosylation Position by Using Negative Ion Electrospray Ionization Tandem Mass Spectrometry. J. Mass Spectrom. JMS 2006, 41, 352–360. [Google Scholar] [CrossRef] [PubMed]
- Saldanha, L.L.; Vilegas, W.; Dokkedal, A.L. Characterization of Flavonoids and Phenolic Acids in Myrcia bella Cambess. Using FIA-ESI-IT-MSn and HPLC-PAD-ESI-IT-MS Combined with NMR. Molecules 2013, 18, 8402–8416. [Google Scholar] [CrossRef] [PubMed]
- Simirgiotis, M.J. Antioxidant Capacity and HPLC-DAD-MS Profiling of Chilean peumo (Cryptocarya alba) Fruits and Comparison with German Peumo (Crataegus monogyna) from Southern Chile. Molecules 2013, 18, 2061–2080. [Google Scholar] [CrossRef] [PubMed]
- Takao, L.K.; Imatomi, M.; Gualtieri, S.C.J. Antioxidant activity and phenolic content of leaf infusions of Myrtaceae species from Cerrado (Brazilian Savanna). Braz. J. Biol. 2015, 75, 948–952. [Google Scholar] [CrossRef] [PubMed]
- Denardin, C.C.; Hirsch, G.E.; Da Rocha, R.F.; Vizzotto, M.A.T.H.; Moreira, J.C.F.; Guma, F.T.C.R.; Emanuelli, T. Antioxidant Capacity and Bioactive Compounds of Four Brazilian Native Fruits. J. Food Drug Anal. 2015, 23, 387–398. [Google Scholar] [CrossRef] [PubMed]
- Fouche, G.; Cragg, G.M.; Pillay, P.; Kolesnikova, N.; Maharaj, V.J.; Senabe, J. In vitro anticancer screening of South African plants. J. Ethnopharmacol. 2008, 119, 455–461. [Google Scholar] [CrossRef] [PubMed]
- Franco, D.M.; Saldanha, L.L.; Silva, E.M.; Nogueira, F.T.S.; Dokkedal, A.L.; Santos, C.; Rolim, D.E.; Almeida, L.F. Effects of leaf extracts of Myrcia guianensis (Aubl.) DC. on growth and gene expression during root development of Sorghum bicolor (L.) moench. Allelopath. J. 2015, 35, 237–248. [Google Scholar]
- Serpeloni, J.M.; Specian, A.F.; Ribeiro, D.L.; Tuttis, K.; Vilegas, W.; Martínez-López, W.; Dokkedal, A.L.; Saldanha, L.L.; Cólus, I.M.; Varanda, E.A. Antimutagenicity and induction of antioxidant defense by flavonoid rich extract of Myrcia bella Cambess. in normal and tumor gastric cells. J. Ethnopharmacol. 2015, 176, 345–355. [Google Scholar] [CrossRef] [PubMed]
- Singh, A.K.; Sharma, N.; Ghosh, M.; Park, Y.H.; Jeong, D.K. Emerging importance of dietary phytochemicals in fight against cancer: Role in targeting cancer stem cells. Crit. Rev. Food Sci. Nutr. 2017, 57, 3449–3463. [Google Scholar] [CrossRef] [PubMed]
- Cowan, M.M. Plant Products as Antimicrobial Agents. Clin. Microbiol. Rev. 1999, 12, 564–582. [Google Scholar] [PubMed]
- Carneiro, N.S.; Alves, C.C.F.; Alves, J.M.; Egea, M.B.; Martins, C.H.G.; Silva, T.S.; Bretanha, L.C.; Balleste, M.P.; Micke, G.A.; Silveira, E.V.; et al. Chemical Composition, Antioxidant and Antibacterial Activities of Essential Oils from Leaves and Flowers of Eugenia klotzschiana Berg (Myrtaceae). Ann. Braz. Acad. Sci. 2017, 89, 1907–1915. [Google Scholar] [CrossRef] [PubMed]
- CISI. Methods for Dilution Antimicrobial Susceptibility Tests for Bacteria That Grow Aerobically; Approved Standard, 9th ed.; CISI: Wayne, PA, USA, 2012; Volume 32. [Google Scholar]
- Pereira, J.V.; Freiresb, I.A.; Castilho, A.R.; da Cunha, M.G.; Alves Hda, S.; Rosalen, P.L. Antifungal potential of Sideroxylon obtusifolium and Syzygium cumini and their mode of action against Candida albicans. Pharm. Biol. 2016, 54, 2312–2319. [Google Scholar] [CrossRef] [PubMed]
- Kalia, K.; Sharma, K.; Singh, H.P.; Singh, B. Effects of extraction methods on phenolic contents and antioxidant activity in aerial parts of Potentilla atrosanguinea Lodd. and quantification of its phenolic constituents by RPHPLC. J. Agric. Food Chem. 2008, 56, 10129–10134. [Google Scholar] [CrossRef] [PubMed]
- Enujiugha, V.N.; Talabi, J.Y.; Malomo, S.A.; Olagunju, A.I. DPPH Radical Scavenging Capacity of Phenolic Extracts from African Yam Bean (Sphenostylis stenocarpa). Food Nutr. Sci. 2012, 3, 7–13. [Google Scholar] [CrossRef]
Sample Availability: Samples of the compounds are not available from the authors. |
Formula [M − H]− | Theoretical Mass [M − H] m/z | Experimental Mass [M − H]− m/z | ∆m (ppm) | MS/MS Fragments m/z | Compound Identification | Myrcia Species |
---|---|---|---|---|---|---|
C7H5O5 | 169.0158 | 169.0162 | 2.36 | 151, 125 | gallic acid | Mb |
C7H11O6 | 191.0561 | 191.0562 | −0.52 | 173, 111 | quinic acid | Mb Mf Mg |
C14H5O8 | 300.9987 | 300.9979 | 0.84 | 257, 229 | ellagic acid | Mf Mb |
C13H15O10 | 331.0671 | 331.0668 | 2.65 | 271, 211, 169, 125 | monogalloylglucose | Mb Mf Mg |
C14H15O10 | 343.0666 | 343.0660 | 1,75 | 191, 169, 125 | monogalloylquinic acid | Mf |
C20H17O14 | 481.0624 | 481.0623 | 0.16 | 301, 275 | HHDP-glucose isomer | Mf |
C20H19O14 | 483.0770 | 483.0775 | −1.03 | 331, 169, 125 | digalloyl-glucose isomer | Mf Mg |
C34H24O22 | 783.0657 | 783.0659 | −0.26 | 481, 301, 191 | bis HHDP-glucose | Mf |
C41H27O26 | 935.0708 | 935.0760 | −5.56 | 783, 481, 451, 301 | bis HHDP-galloylglucose (casuarinin) | Mf |
C48H29O30 | 1085.0720 | 1085.0738 | −1.66 | 935, 783, 301 | eucalbanin A/cornusin B isomer | Mf |
Formula [M − H]− | Theoretical Mass [M − H] m/z | Experimental Mass [M − H]− m/z | ∆m (ppm) | MS/MS Fragments m/z | Compound Identification | Myrcia Species |
---|---|---|---|---|---|---|
C15H9O7 | 301.0348 | 301.0348 | 0.01 | 257, 179, 151 | quercetin | Mf Mb |
C15H9O8 | 317.0303 | 317.0304 | −0.32 | 299, 179, 151, 137, 107 | myricetin | Mb Mg Mf |
C20H17O11 | 433.0768 | 433.0765 | 0.69 | 300, 271, 151, | quercetin–3-O-β-d-xylopyranoside | Mf Mb |
C21H19O11 | 447.0927 | 447.0932 | −0.28 | 316, 287, 271, 179 | quercetin-3-O-β-rhamnoside | Mf Mg Mb |
C20H17O12 | 449.0718 | 449.0720 | −1.12 | 316, 271,179 | myricetin-3-O-arabinoside | Mf Mg |
C21H19O12 | 463.0877 | 463.0880 | −0.65 | 317, 271, 179 | myricetin-3-O-rhamnoside | Mf Mb Mg |
C21H19O13 | 479.0821 | 479.0821 | 0.01 | 316, 271, 179, 151 | myricetin-3-O-β-glucoside | Mf Mg |
C27H21O15 | 585.0886 | 585.0877 | 1.54 | 433, 301, 191 | Quercetin-O-(O-galloyl)pentoside | Mf Mb |
C27H21O16 | 601.0835 | 601.0823 | 2.00 | 449, 316, 271, 179 | Myricetin-O-(O-galloyl)arabinoside | Mf Mb |
C28H23O16 | 615.0947 | 615.0981 | 0.98 | 463, 317, 301, 179, 151 | myricetin rhamnopyranoside | Mf Mb Mg |
C28H23O17 | 631.0930 | 631.0928 | 0.32 | 479, 316, 191, 169, 151 | Myricetin galloyl galactoside | Mf Mg |
C28H31O17 | 639.1567 | 639.1553 | 2.19 | 447, 300, 191 | quercetin-3-O-galloyldeoxipentose | Mb |
C28H31O18 | 655.1516 | 655.1510 | 0.92 | 463, 316, 191 | myricetin galloyl rhamnopyranoside | Mb Mf Mg |
Reagent/Extract | Phenol Content a | Flavonoid Content a | DPPH (µg/mL) |
---|---|---|---|
mgGAc/gextract | mgQ/gextract | EC50 | |
Gallic Acid | 2.03 ± 0.02 a | ||
Quercetin | 2.96 ± 0.17 a | ||
M. bella | 215.39 ± 0.01 a | 20.24 ± 0.48 a | 13.8 ± 0.53 b |
M. fallax | 218.19 ± 0.81 a | 25.92 ± 0.50 b | 8.61 ± 0.22 c |
M. guianensis | 71.54 ± 0.01 b | 19.17 ± 0.30 a | 16.2 ± 0.80 d |
MCF-7 | NCI-ADR/RES | 786-0 | NCI-H460 | PC-3 | HT-29 | K562 | |
---|---|---|---|---|---|---|---|
DOX | 3.30 | 6.60 | 2.67 | 0.90 | 5.85 | 3.90 | 20.48 |
M. bella | 30.50 | 132.48 | 128.69 | 139.82 | 94.23 | 63.58 | 19.66 |
M. fallax | >250 | >250 | 245 | 110.95 | 190.78 | 151.11 | 43.86 |
M. guianensis | 43.32 | >250 | 209.83 | 193.29 | 94.73 | 170.34 | 7.45 |
© 2018 by the authors. Licensee MDPI, Basel, Switzerland. This article is an open access article distributed under the terms and conditions of the Creative Commons Attribution (CC BY) license (http://creativecommons.org/licenses/by/4.0/).
Share and Cite
Dos Santos, C.; Galaverna, R.S.; Angolini, C.F.F.; Nunes, V.V.A.; De Almeida, L.F.R.; Ruiz, A.L.T.G.; De Carvalho, J.E.; Duarte, R.M.T.; Duarte, M.C.T.; Eberlin, M.N. Antioxidative, Antiproliferative and Antimicrobial Activities of Phenolic Compounds from Three Myrcia Species. Molecules 2018, 23, 986. https://doi.org/10.3390/molecules23050986
Dos Santos C, Galaverna RS, Angolini CFF, Nunes VVA, De Almeida LFR, Ruiz ALTG, De Carvalho JE, Duarte RMT, Duarte MCT, Eberlin MN. Antioxidative, Antiproliferative and Antimicrobial Activities of Phenolic Compounds from Three Myrcia Species. Molecules. 2018; 23(5):986. https://doi.org/10.3390/molecules23050986
Chicago/Turabian StyleDos Santos, Catarina, Renan S. Galaverna, Celio F. F. Angolini, Vania V. A. Nunes, Luiz F. R. De Almeida, Ana L. T. G. Ruiz, João E. De Carvalho, Regina M. T. Duarte, Marta C. T. Duarte, and Marcos N. Eberlin. 2018. "Antioxidative, Antiproliferative and Antimicrobial Activities of Phenolic Compounds from Three Myrcia Species" Molecules 23, no. 5: 986. https://doi.org/10.3390/molecules23050986