An Automated Three-Dimensional Bone Pose Tracking Method Using Clinical Interleaved Biplane Fluoroscopy Systems: Application to the Knee
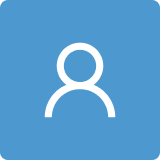
Round 1
Reviewer 1 Report
The paper matches the Journal's items dealing with kinematic measurement investigations, and specifically focusing on the presentation of an automated model-based interleaved biplane fluoroscopy image tracking scheme (MIBFT) to measure non-invasively the 3D kinematics of tibiofemoral motion. The proposed method permits to achieve fully automated image registration for a complete image set.
A comparison to other approaches to existing clinical imaging systems is included for the erroneous skeletal kinematics estimation to be reduced, obtaining accuracy of the measured bone poses by incorporating information of adjacent image frames. The performance of the new model-based tracking method is evaluated experimentally on a knee specimen.
In the paper the system used is described enough in detail with the precise distinction of the stages (three stages so distinguished: 2D/2D template registration; frame interpolation and biplane 3D/2D image registration) and then evaluated through the simulated knee-motion experiment on the knee specimen. The reconstruction of the tools used in the cadaveric experiment for all numerical computations is provided.
The MIBFT managed to reproduce skeletal poses and tibiofemoral kinematics reporting the root- mean-squared (Rms) errors of the skeletal pose parameters and showing the influences of rotation speeds on the pose errors. Bias, precision and Rms errors are reported in the comparative analysis.
The authors outline that their proposed system proved its effectiveness for dynamic tibiofemoral kinematics measurements (achieving less than 0.4 mm Rms error in measuring tibiofemoral translation and 0.8° Rms error in measuring rotation).
The results are enough discussed advancing a useful employment of the clinical imaging system for the noninvasive and precise examination of dynamic joint functions and kinematics and its further application in clinical practice and biomechanical research.
The paper presents sufficiently interesting results for future research in the field defining the assumptions for other clinical applications. However, the paper can just be improved before publication. Thus, the authors are recommended to follow some suggestions for the revision work of their final manuscript:
- Though the argumentation on the topic of the study is sufficiently presented, the aim of the work should be better specified in relation to the achievable results rather than generally indicated in the Introduction section as “to propose and evaluate a new automated model-based tracking scheme for the noninvasive measurement of the 3D kinematics of tibiofemoral motion”.
- For clarity of presentation, in order to facilitate an immediate comprehension of section 2, the authors should start the section with a brief description of the procedure used and illustrated in the following subsections.
- The authors are also invited to take into consideration the possibility to insert their analysis on the system evaluation into a separate section rather than in the subsection 2.4 Performance evaluation.
- All figures must be checked carefully so as to be clearly readable. The figure legends should have different margins (left and right) with respect to the manuscript. Tables should be as small as possible. Please reduce the dimensions of the tables included.
- The conclusion should also refer more specifically to other possible applications to different areas of interest.
- As far as the English language is concerned, there aren't many particular errors in the manuscript. A correction of tenses in the Introduction and section 4 is appreciable as much as an improvement in the formulation of some sentences in the paper (i.e. "He had provided written informed consent but the authors had no access to information that could identify the donor", in section 2). It is also suggested to avoid often repeating the same vocabulary all over the text.
Please check and correct the following sentences:
Abstract
- Compared to those of the reported model-based tracking techniques with custom-made synchronous biplane fluoroscopy, the MIBFT-determined bias, precision and Rms error were comparable.
Introduction
- Quantifying the subject-and task-specific rigid-body kinematics and cartilage contact arthrokinematics of the tibiofemoral joint are crucial for improving the understanding the biomechanics and pathomechanics of the joint and surrounding soft tissues [2,3],
- The application of X-ray fluoroscopic imaging enables less restricted kinematic measurements during functional tasks, especially mobile imaging systems that were introduced recently
- While MCPI improved translation accuracy over single-plane model-based tracking, it appeared to not substantially ameliorate the rotational accuracy [40]
Section 2
- Using the segmented bone regions, surface bone models were also constructed using a marching cubes algorithm [45], which were used to create anatomical frames with an automatic determination method [46].
- The length of the femur and tibia of the fresh-frozen knee specimen was approximately 260 mm and 290 mm, respectively.
Section 4
- This thus diminishes the pose parameter search space in the subsequent 3D/2D image registration, increasing the probability of finding the optimal solution, especially for those tasks involving greater between-frame displacement (Figure 7)
Conclusions
- A new fully automated model-based interleaved biplane fluoroscopy image tracking scheme in conjunction with clinical asynchronous biplane fluoroscopy was proposed and evaluated for its performance on dynamic tibiofemoral kinematics measurements.
Comments for author File: Comments.pdf
Author Response
Response to Reviewer 1 Comments
Point 1: Though the argumentation on the topic of the study is sufficiently presented, the aim of the work should be better specified in relation to the achievable results rather than generally indicated in the Introduction section as “to propose and evaluate a new automated model-based tracking scheme for the noninvasive measurement of the 3D kinematics of tibiofemoral motion”.
Response 1: Thanks for the reviewer’s invaluable suggestion on the article. To address the comment, we have revised the statement regarding the study aims, which now reads “the present work aimed to develop a new automated model-based tracking scheme for the noninvasive measurement of the 3D kinematics of tibiofemoral motion with an accuracy comparable to those using custom-made synchronous biplane X-ray imaging system. A motion experiment with a knee specimen was performed to evaluate the accuracy of the new model-based tracking method on the determination of 3D bone poses and joint kinematics during dynamic tibiofemoral motions. The influence of the joint rotation speeds on the measurement errors was also quantified. “. (Lines 94-100, Pages 2-3)
Point 2: For clarity of presentation, in order to facilitate an immediate comprehension of section 2, the authors should start the section with a brief description of the procedure used and illustrated in the following subsections.
Response 2: A new sub-section “overview” has been added to provide a brief description of the procedure developed in the present study, which reads “The dynamic tibiofemoral motions are recorded using a clinical asynchronous biplane fluoroscopy imaging system. The CT data of the same joint is also acquired and used to create volumetric models of the femur and tibia. The 3D pose parameters of the bone in each fluoroscopic frame are determined using the new model-based tracking scheme composed of three major stages, namely (i) 2D/2D template registration; (ii) motion-compensated frame interpolation; and (iii) biplane 3D/2D image registration. The registered bone poses for the entire image sequence are then used to compute 3D kinematics of the tibiofemoral joint. A motion experiment using a cadaveric knee joint was performed to evaluate the measurement accuracy of the proposed method. Detailed illustrations of the model-based tracking and motion experiment are provided in the following subsections.”. (Lines 103-111, Page 3)
Point 3: The authors are also invited to take into consideration the possibility to insert their analysis on the system evaluation into a separate section rather than in the subsection 2.4 Performance evaluation.
Response 3: Following the suggestion by the reviewer, we have separated the original subsection 2.4 into two new subsections 2.5 In vitro motion experiment and 2.6 Evaluation of MIBFT to describe separately the motion experiment and analysis procedures for the experiment.
Point 4: All figures must be checked carefully so as to be clearly readable. The figure legends should have different margins (left and right) with respect to the manuscript. Tables should be as small as possible. Please reduce the dimensions of the tables included.
Response 4: To address the comment, we have enlarged the size of text in Figure 2, 6, 7, 8, and 9 to improve the readability. The margin and size of the figure legends are corrected following the format of the journal. The dimension of the table is also reduced as much as possible. Please see the revised figures and tables.
Point 5: The conclusion should also refer more specifically to other possible applications to different areas of interest.
Response 5: To address the comment, we have added a new description regarding the potential application of the proposed model-based tracking scheme in the field of image-guided interventions, which reads “The presented approach may be also integrated into image-guided intervention procedures to spatially align the pre-interventional image data with the intra-intervention data.” (Lines 467-469, Page 16)
Point 6: As far as the English language is concerned, there aren't many particular errors in the manuscript. A correction of tenses in the Introduction and section 4 is appreciable as much as an improvement in the formulation of some sentences in the paper (i.e. "He had provided written informed consent but the authors had no access to information that could identify the donor", in section 2). It is also suggested to avoid often repeating the same vocabulary all over the text.
Please check and correct the following sentences:
Abstract
- Compared to those of the reported model-based tracking techniques with custom-made synchronous biplane fluoroscopy, the MIBFT-determined bias, precision and Rms error were comparable.
Introduction
- Quantifying the subject-and task-specific rigid-body kinematics and cartilage contact arthrokinematics of the tibiofemoral joint are crucial for improving the understanding the biomechanics and pathomechanics of the joint and surrounding soft tissues [2,3],
- The application of X-ray fluoroscopic imaging enables less restricted kinematic measurements during functional tasks, especially mobile imaging systems that were introduced recently
- While MCPI improved translation accuracy over single-plane model-based tracking, it appeared to not substantially ameliorate the rotational accuracy [40]
Section 2
- Using the segmented bone regions, surface bone models were also constructed using a marching cubes algorithm [45], which were used to create anatomical frames with an automatic determination method [46].
- The length of the femur and tibia of the fresh-frozen knee specimen was approximately 260 mm and 290 mm, respectively.
Section 4
- This thus diminishes the pose parameter search space in the subsequent 3D/2D image registration, increasing the probability of finding the optimal solution, especially for those tasks involving greater between-frame displacement (Figure 7)
Conclusions
- A new fully automated model-based interleaved biplane fluoroscopy image tracking scheme in conjunction with clinical asynchronous biplane fluoroscopy was proposed and evaluated for its performance on dynamic tibiofemoral kinematics measurements.
Response 6: We have checked carefully and corrected the sentences indicated by the reviewer. Please see the revised sentences:
- The MIBFT-determined bias, precision and Rms error were comparable to those of the reported model-based tracking techniques using custom-made synchronous biplane fluoroscopy. (Lines 38-40, Page 1)
- With quantified data of rigid-body kinematics and cartilage contact arthrokinematics of the tibiofemoral joint, both subject- and task-specific biomechanics and pathomechanics can be explored further. (Lines 47-49, Page 2)
- The application of standard X-ray fluoroscopy or newly developed mobile fluoroscopic imaging systems enable more dynamic kinematic measurements during functional tasks [21,22]. (Lines 65-66, Page 2)
- While the MCPI improved translation accuracy over single-plane model-based tracking, rotational errors were not significantly improved [40]. (Lines 85-87, Page 2)
- Surface bone models were constructed with a marching cubes algorithm [45] and segmented bone regions. Anatomical frames were then created using an automatic determination method [46]. (Lines 132-134, Page 3)
- The knee specimen used in the current in vitro experiment was obtained from a male donor who received a below-hip amputation for disorders other than the knee joint and had provided written informed consent. Access to information identifying the donor was not provided to the authors. (Lines 230-233, Page 8)
- Lengths of the femur and tibia of the fresh-frozen knee specimen were approximately 260 mm and 290 mm, respectively. (Lines 236-237, Page 8)
- This diminishes the search space of pose parameters required in the subsequent 3D/2D image registration and therefore increases the probability of finding the optimal solution, allowing for measurement of faster motions involving a greater between-frame bone displacement (Figure 7). (Lines 438-441, Page 15)
- A new fully automated model-based tracking scheme in conjunction with clinical asynchronous biplane fluoroscopy has been developed and evaluated for the accuracy on the measurement of dynamic tibiofemoral motions. (Lines 460-462, Page 16)
Author Response File: Author Response.docx
Reviewer 2 Report
Automated model-based interleaved biplane fluoroscopy image tracking scheme by incorporating information of adjacent image frames is proposed. The proposed method is evaluated by a cadaveric study conducted on a knee specimen. The proposed method skeletal posed and tibiofemoral kinematics are shown to be in good agreement with the standard reference kinematics obtained from an optical motion capture system. The root-mean-square (RMS) errors of the skeletal pose parameters for translation range from 0.11 mm to 0.35 mm. For rotation, the RMS is found to be between 0.18 degrees and 0.49 degrees. The influence of rotational speed on the translational and rotational motions is reported to be 0.32 mm and 0.26 degrees, respectively. The proposed method's results in terms of bias, precision, and RMS error are comparable to the reported model-based tracking techniques with custom-made synchronous biplane fluoroscopy.
Comments:
- Line 247: The tibia was moved manually while the femur was kept immobile. The rotational speeds in cycles per second are recorded as 0.25, 0.375, 0.5, 0.625, and 0.75. How could you measure the rotational speed? Was the rotational speed constant?
- Figure 2: The texts in the figure are too small.
- Figures 6, 7, 8, and 9: the subplots fonts of the axis, labels, and legends are small.
Author Response
Response to Reviewer 2 Comments
Point 1: Line 247: The tibia was moved manually while the femur was kept immobile. The rotational speeds in cycles per second are recorded as 0.25, 0.375, 0.5, 0.625, and 0.75. How could you measure the rotational speed? Was the rotational speed constant?
Response 1: The rotation speeds in the unit of “cycles/s” were prescribed speed conditions planning in the experiment and controlled by the operator following the cadence from a metronome (Lines 259-261, Page 8), and thus the rotation speeds were not ideally constant during the image data acquisition. Therefore, in order to quantify the exact rotation speeds, the tibiofemoral motions measured by the motion capture system were used to calculate the rotation speeds. This information has been added in the manuscript for better clarity, which now reads “The rotation speeds of the tibiofemoral motions were also derived using the quantified flexion angles at each time instants.” (Lines 285-286, Page 9). The exact quantified results had been given in the section “Result” (Lines 322-326, Page 10).
Point 2: Figure 2: The texts in the figure are too small.
Response 2: Following the suggestion, we have revised Figure 2 by enlarging the size of the text in the figure.
Point 3: Figures 6, 7, 8, and 9: the subplots fonts of the axis, labels, and legends are small.
Response 3: To address the comment, Figures 6,7,8 and 9 are revised. The font size of the axis, labels and figure legends are enlarged.
Author Response File: Author Response.docx